
More than two decades before the first exoplanet was discovered, an experiment was performed using a moving flame and liquid mercury that could hold the key to habitability on tidally locked worlds.
The paper was published in a 1969 edition of the international journal, Science, by researchers Schubert and Whitehead. The pair reported that when a Bunsen flame was rotated beneath a cylindrical container of mercury, the liquid began to flow around the container in the opposite direction at speeds up to four times greater than the rotation of the flame. The scientists speculated that such a phenomenon might explain the rapid winds on Venus.
On the Earth, the warm equator and cool poles set up a pressure difference that creates our global winds. These winds are deflected westward by the rotation of the planet (the so-called Coriolis force) promoting a zonal (east-west) air flow around the globe. But what would happen if our planet’s rotation slowed? Would our winds just cycle north and south between the equator and poles?

Such a slow-rotating scenario may be the lot of almost all rocky exoplanets discovered to date. Planets such as the TRAPPIST-1 system and Proxima Centauri-b all orbit much closer to their star than Mercury, making their faint presence easier to detect but likely resulting in tidal lock. Like the moon orbiting the Earth, planets in tidal lock have one side permanently facing the star, creating a day that is equal to the planet’s year.
The dim stars orbited by these planets can mean they receive a similar level of radiation as the Earth, placing them within the so-called “habitable zone.” However, tidal lock comes with the risk of horrific atmospheric collapse. On the planet side perpetually facing away from the star, temperatures can drop low enough to freeze an Earth-like atmosphere. The air from the dayside would then rush around the planet to fill the void, freezing in turn and causing the planet to lose its atmosphere even within the habitable zone.
The only way this could be prevented is if winds circulating around the planet could redistribute the heat sufficiently to prevent freeze-out. But without a strong Coriolis force from the planet’s rotation, can such winds exist?
A planet whose wind speeds exceed the rotation speed of the planet is said to have a “super-rotating” atmosphere. Global climate models of tidally locked planets have suggested that temperate conditions might be maintained by winds circulating between the night and day side in the same way as the Earth’s winds are generated between the equator and poles.
However, global climate models are extremely tricky, being computationally expensive and sensitive to a multitude of factors that are unmeasurable for exoplanets. As a result, it has not been possible to test if the climates produced by the computer could really exist, leaving the fate of tidally locked worlds uncertain.

But there is a slowly rotating planet where one mechanism for super rotation can be explored. Venus is the only other Earth-sized planet we can reach by spacecraft and the planet has super rotating winds whose origin has been hotly debated for decades.
While Venus is not in tidal lock with the sun, its rotation is extremely slow. Our neighboring world takes 225 days to orbit the sun and rotates once every 243 Earth days, making the Venusian day (one rotation) longer than its year.
The planet’s thick carbon dioxide atmosphere provides Venus with the most powerful greenhouse effect in the solar system. This prevents nights on Venus from freezing and the planet maintains a fairly uniform surface temperature that is hot enough to melt lead. Yet despite the lack of a temperature gradient to drive winds, the upper atmosphere of Venus is a blistering gale. Winds whip around the planet with speeds that exceed 100 m/s (224 mph), traveling sixty times faster than the surface rotation. What could be driving this weather system?

It is a question that returns us to the Bunsen flame experiment. Schubert and Whitehead speculated that the sun could replace the Bunsen flame in driving the Venusian winds rapidly around the planet. However, skeptics to this claim argued that the sun could only influence the cloud tops of Venus, whereas super-rotation had been observed to extend much deeper into the planet’s thick cloak of gases.
The alternative theory was that small contrasts in heat on the planet’s surface could set up circulations to drive the super-rotating winds. A challenge here was that such models appeared very sensitive to the exact starting conditions, suggesting that Venus’s super-rotating winds were a rare outcome for the planet. If true, the same mechanism was not likely to be acting on tidally locked worlds around other stars.
At the end of last year, a paper in the Astrophysical Journal Supplement Series was published that provided a heap of new data about Venus’s atmosphere. The research was led by Javier Peralta, a postdoctoral fellow at the Japan Aerospace Exploration Agency (JAXA). Using data from JAXA’s Venus orbiter, Akatsuki, Peralta had painstakingly tracked the Venusian winds.
The IR2 camera onboard Akatsuki captures images in the infrared at a wavelength of 2.26 microns. On the dayside of Venus, clouds in the upper atmosphere sitting at 60 – 70 km (37 – 44 miles) above the planet’s surface strongly reflect ultraviolet and infrared from the Sun. However, the nightside illumination comes from infrared heat emanating from Venus’s hot surface. This is partially blocked by clouds deeper in the atmosphere at altitudes between 48 – 60 km (30 – 37 miles). As the clouds have a varying transparency to this infrared glow, their shapes become visible when viewed through Akatsuki’s IR2 camera. It was these deeper, nightside clouds, that Peralta tracked.
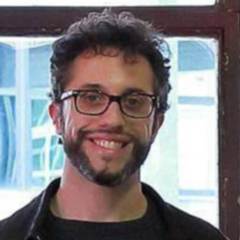
By comparing results from 2,947 wind measurements, Peralta spotted a pattern. The winds acceleration was tied to the position of the sun, suggesting that the giant Bunsen-flame of our nearest star was indeed driving super-rotation deep in the Venusian atmosphere. It was a result that suggested this super-rotation mechanism could be common on many more planets, as it required only the heat from the star.
Yet, Peralta was quick to note that this was not a “case closed” for the Venusian winds. While they had not detected a north-south component to the winds that would have supported the alternative theory of a surface-driven origin for the super-rotating, it might have just been below the level they could detect.
“This was one reason why we made our wind measurements publicly available,” Peralta said. “Sharing measurements is critical nowadays since the new generation of computer models are able to incorporate this observational data to predict how an atmosphere will evolve.”
Peralta hopes that results from Venus can used with climate models for slow rotating worlds, helping scientists understand both our nearest neighbor and the conditions that might be present on tidally locked planets by providing comparative measurements for at least one type of super rotation generation.
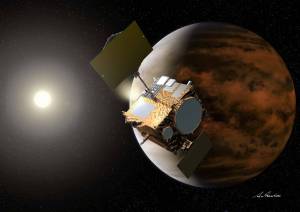
As well as using results from Akatsuki, Peralta also looked at wind speed measurements from previous missions and ground observations of Venus. Comparing data since the late 1970s, his team noted a variation in the recorded wind speeds. While it is challenging to compare results from different instruments (which have different error estimates), this might suggest that the Venusian weather pattern has varied over time scales of decades.
Such variation could also support the sun being the main driver for the winds. Changes in the cloud’s reflectivity would alter how much solar radiation is absorbed, adjusting the efficiency of this driving force. If true, this could be used to calibrate models further for different reflectivity conditions.
Peralta’s results underline the importance of our solar system in understanding exoplanets. The comparison of the weird and wonderful climates among our neighboring worlds can help us explore the next Earth-sized discovery and these worlds are within the reach of our spaceships.