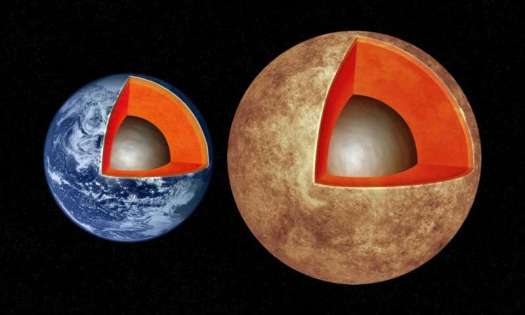
The quest to find habitable — and perhaps inhabited — planets and moons beyond Earth focuses largely on their location in a solar system and the nature of its host star, the eccentricity of its orbit, its size and rockiness, and the chemical composition of its atmosphere, assuming that it has one.
Astronomy, astrophysics, cosmochemistry and many other disciplines have made significant progress in characterizing at least some of the billions of exoplanets out there, although measuring the chemical makeup of atmospheres remains a immature field.
But what if these basic characteristics aren’t sufficient to answer necessary questions about whether a planet is habitable? What if more information — and even more difficult to collect information — is needed?
That’s the position of many planetary scientists who argue that the dynamics of a planet’s interior are essential to understand its habitability.
With our existing capabilities, observing an exoplanet’s atmospheric composition will clearly be the first way to search for signatures of life elsewhere. But four scientists at the Carnegie Institution of Science — Anat Shahar, Peter Driscoll, Alycia Weinberger, and George Cody — argued in a recent perspective article in Science that a true picture of planetary habitability must consider how a planet’s atmosphere is linked to and shaped by what’s happening in its interior.
They argue that on Earth, for instance, plate tectonics are crucial for maintaining a surface climate where life can fill every niche. And without the cycling of material between the planet’s surface and interior, the convection that drives the Earth’s magnetic field would not be possible and without a magnetic field, we would be bombarded by cosmic radiation.
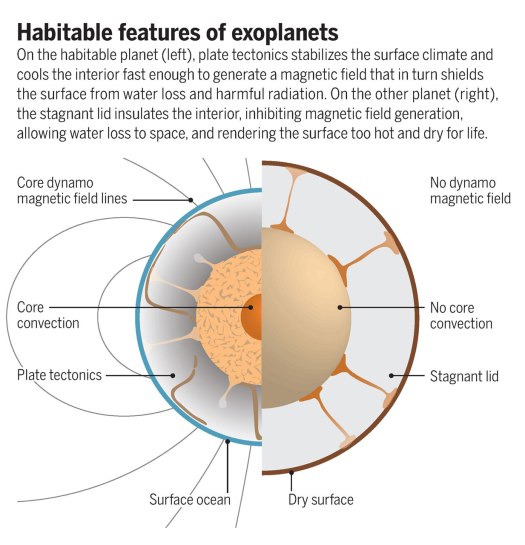
“The perspective was our way to remind people that the only exoplanet observable right now is the atmosphere, but that the atmospheric composition is very much linked to planetary interiors and their evolution,” said lead author Shahar, who is trained in geological sciences. “If there is a hope to one day look for a biosignature, it is crucial we understand all the ways that interiors can influence the atmospheric composition so that the observations can then be better understood.”
“We need a better understanding of how a planet’s composition and interior influence its habitability, starting with Earth,” she said. “This can be used to guide the search for exoplanets and star systems where life could thrive, signatures of which could be detected by telescopes.”
It all starts with the formation process. Planets are born from the rotating ring of dust and gas that surrounds a young star.
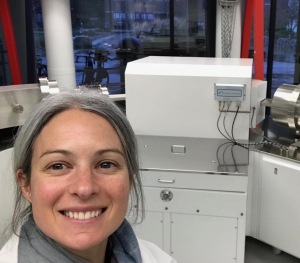
The elemental building blocks from which rocky planets form–silicon, magnesium, oxygen, carbon, iron, and hydrogen–are universal. But their abundances and the heating and cooling they experience in their youth will affect their interior chemistry and, in turn, defining factors such ocean volume and atmospheric composition.
“One of the big questions we need to ask is whether the geologic and dynamic features that make our home planet habitable can be produced on planets with different compositions,” Carnegie planetary scientist Peter Driscoll explained in a release.
In the next decade as a new generation of telescopes come online, scientists will begin to search in earnest for biosignatures in the atmospheres of rocky exoplanets. But the colleagues say that these observations must be put in the context of a larger understanding of how a planet’s total makeup and interior geochemistry determines the evolution of a stable and temperate surface where life could perhaps arise and thrive.
“The heart of habitability is in planetary interiors,” concluded Carnegie geochemist George Cody.
Our knowledge of the Earth’s interior starts with these basic contours: it has a thin outer crust, a thick mantle, and a core the size of Mars. A basic question that can be asked and to some extent answered now is whether this structure is universal for small rocky planets. Will these three layers be present in some form in many other rocky planets as well?
Earlier preliminary research published in the The Astrophysical Journal suggests that the answer is yes – they will have interiors very similar to Earth.
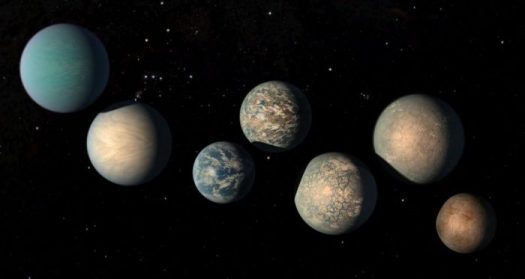
“We wanted to see how Earth-like these rocky planets are. It turns out they are very Earth-like,” said lead author Li Zeng of the Harvard-Smithsonian Center for Astrophysics (CfA
To reach this conclusion Zeng and his co-authors applied a computer model known as the Preliminary Reference Earth Model (PREM), which is the standard model for Earth’s interior. They adjusted it to accommodate different masses and compositions, and applied it to six known rocky exoplanets with well-measured masses and physical sizes.
They found that the other planets, despite their differences from Earth, all should have a nickel/iron core containing about 30 percent of the planet’s mass. In comparison, about a third of the Earth’s mass is in its core. The remainder of each planet would be mantle and crust, just as with Earth.
“We’ve only understood the Earth’s structure for the past hundred years. Now we can calculate the structures of planets orbiting other stars, even though we can’t visit them,” adds Zeng.
The model assumes that distant exoplanets have chemical compositions similar to Earth. This is reasonable based on the relevant abundances of key chemical elements like iron, magnesium, silicon, and oxygen in nearby systems. However, planets forming in more or less metal-rich regions of the galaxy could show different interior structures.
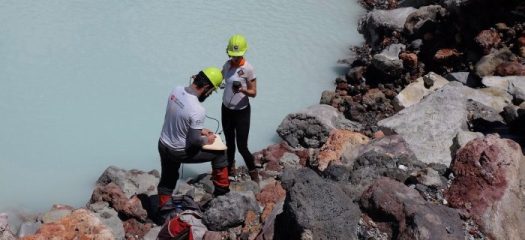
While thinking about exoplanetary interiors—and some day finding ways to investigate them — is intriguing and important, it’s also apparent that there’s a lot more to learn about role of the Earth’s interior in making the planet habitable.
In 2017, for instance, an interdisciplinary group of early career scientists visited Costa Rica’s subduction zone, (where the ocean floor sinks beneath the continent) to find out if subterranean microbes can affect geological processes that move carbon from Earth’s surface into the deep interior.
According to their new study in Nature, the answer is yes.
The study shows that microbes consume and trap a small but measurable amount of the carbon sinking into the trench off Costa Rica’s Pacific coast. The microbes may also be involved in chemical processes that pull out even more carbon, leaving cement-like veins of calcite in the crust.
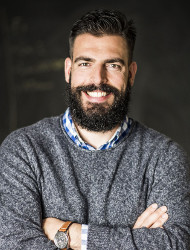
In all, microbes and calcite precipitation combine to trap about 94 percent of the carbon squeezed out from the edge of the oceanic plate as it sinks into the mantle during subduction. This carbon remains naturally sequestered in the crust, where it cannot escape back to the surface through nearby volcanoes in the way that much carbon ultimately recycles.
These unexpected findings have important implications for how much carbon moves from Earth’s surface into the interior, especially over geological timescales. The research is part of the Deep Carbon Observatory’s Biology Meets Subduction project.
Overall, the study shows that biology has the power to affect carbon recycling and thereby deep Earth geology.
“We already knew that microbes altered geological processes when they first began producing oxygen from photosynthesis,” said Donato Giovannelli of University of Naples, Italy (and who I knew from time spent at the Earth-Life Science Institute Tokyo.) He is a specialist in extreme environments and researches what they can tell us about early Earth and possibly other planets.
“I think there are probably even more ways that biology has had an outsized impact on geology, we just haven’t discovered them yet.”
The findings also shows, Giovanelli told me, that subsurface microbes might have a similarly outsized effect on the composition and balancing of atmospheres—“hinting to the possibility of detecting the indirect effect of subsurface life through atmosphere measurements of exoplanets,” he said.
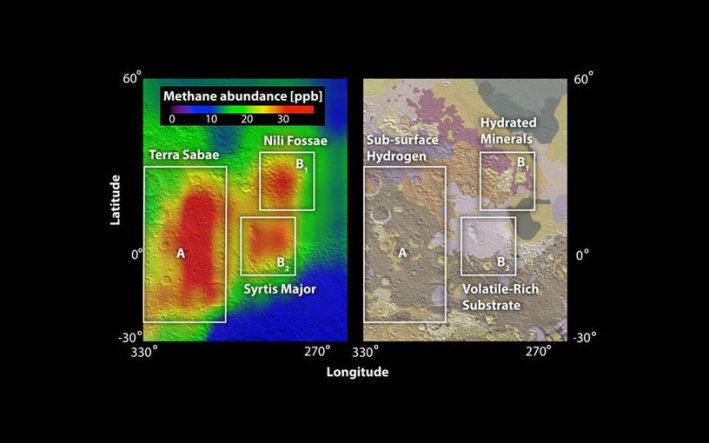
This idea that subsurface life on distant planets could be identified by their byproducts in the atmosphere has just taken on a new immediacy with findings from the Curiosity rover that high levels of the gas methane had recently been detected on Mars. Earlier research had suggested that Mars had some subsurface methane, but the amount appeared to be quite minimal — except as detected once back in 2003 by NASA scientists.
None of the researchers now or in the past have claimed that they know the origin of the methane — whether it is produced biologically or through other planetary processes. But on Earth, some 90 percent of methane comes from biology — bacteria, plants, animals.
Could, then, these methane plumes be a sign that life exists (or existed) below the surface of Mars? It’s possible, and highlights the great importance of what goes on below the surface of planets and moons.
One Reply to “”
Comments are closed.